Photovoltaics are broken down into three generations and are divided into different subtypes based on their material properties. The first and second generations of cells have issues regarding high production costs and less efficiency.
Recent Advancements in Solar Panel Technology
Dr. Raj Shah , Ms. Salowa Siddique, Ms. Mrinaleni Das | Koehler Instrument
Since the beginning of the Industrial Revolution, greenhouse gases have been increasing rapidly due to fossil carbon dioxide emissions. In 2020, China, India, the United States, Japan, and other emerging economies remained the world’s largest carbon dioxide emitters. Together they account for 49.5% of the population, 61.8% of the global Gross Domestic Product, 65.2% of total global fossil fuel consumption (BP, 2021(3)), and 66.7% of total global fossil carbon dioxide emissions [1]. With growing awareness of climate change, the importance of transitioning to cleaner and more sustainable energy sources has increased. Along with having combinations of economic, environmental, and social benefits, solar power does not emit greenhouse gases or harmful byproducts, making it more demandable. Solar power seems much more viable than the many renewable energy options available due to its abundance, regional variability, continuous energy supply, reduction of carbon dioxide emissions, and lower cost of power generation [1]. Thus, there has been an increase in the market of the solar power industry.
The Rise of solar power
Over the years, advancements have been made in solar energy technology. The improvements and widespread adaptation mean solar power is cheaper and more efficient. About 4% of all energy production in the United States comes from solar power, nearly 80 times as much as a decade ago. It accounts for 54% of all new generating capacity. As a result, the solar industry has set a goal to reach 30% of all energy generation by 2030 [2]. Currently, the US is the world's second-largest producer of solar power, with 108.7 GWdc (2021) installed capacity. Since 2008, the production of solar power capacity has grown from 0.34 to 62.4 GWdc showing a 75 fold increase in less than a decade [3]. The United States is emerging as a leading producer of solar power and this article discusses the future prospects of the future solar market based on recent statistics.This article also sheds light on the growing importance of solar power and achieving net zero emissions by 2050. The United States is categorized into five tiers based on its solar potential using an analytical hierarchy process and regression analysis [3]. Figure 1 shows the energy mix in the United States from 2019 and it can be observed that fossil fuels remained dominant. However, projections show that solar will account for 48% of RE generation in 2050 compared to the present 9% [3].
Figure 1: Primary energy sources in the USA (2019) [3].
Three promising solar power technologies
Photovoltaics have become a major contributor to the ongoing energy transition but much improvement is needed in achieving higher efficiency and reducing optical, quantum, and electrical losses of the solar cells. Reducing losses of any kind requires different, often advanced, methods of cell manufacturing and photovoltaic module production. Photovoltaics are broken down into three generations and are divided into different subtypes based on their material properties. The first and second generations of cells have issues regarding high production costs and less efficiency. For example, Monocrystalline solar cells from the first generation are relatively more expensive than polycrystalline ones. And, a major shortcoming of the Amorphous Silicon PV cells is that in labs, the maximum efficiency reached is around 12%. The value degrades largely on a commercial scale which is between 4 to 6% [4]. There has been a lot of attention in the third generation cells, which include Dye-sensitized solar cells and the efficiency trends show that the efficiency will increase in upcoming years.
Dye Sensitized solar cells (DSSCs): DSSCs are a subclass of thin film solar cells and a great alternative to silicon solar cells due to their less complicated production process and do not hinder urban or other commercial applications. It can harvest light efficiently, reduce recombination reactions, improve charge transport ability, and increase dye pickup. A schematic diagram of a DSSC is shown below in Figure 2.
Figure 2: Components of a Dye-sensitized solar cell [5].
Each DSSC component contributes to the solar cell's photocurrent efficiency. The choice of material and structure is crucial since it determines the current-voltage characteristics. A TiO2 nanoparticle structure with ruthenium-based dye achieved an overall lab efficiency of over 12-14%. The photoanode is a crucial component of the DSSCs thus the material has to be selected carefully. TiO2 was the most effective material for photoanode due to its cost-effectiveness, good stability, easy availability, compatible optical, electronic properties, and nontoxicity. Another studied photoanode ZnO had similar work functions as TiO2 but had increased career mobility. ZnO also helped with dye/electrolyte sealing, which means the leakage of liquids in solar devices decreases the lifetime of DSSCs and is unreliable for commercialization. Employing ZnO improved conversion efficiency from 1.2% to 4.5%. The only drawback of using ZnO as a photoanode for a DSSC is that its quick corrosion to acidic environments develops dye aggregates and limits its performance. Thus, TiO2 nanoparticles of 10-20nm and a surface area of 50–100 m2/g have been used in most commercial DSSCs. However, these TiO2 nanoparticle layers show low light scattering ability due to smaller particle size than incident light's wavelength. [5] So based on the results it can be claimed that ZnO works best as dye/electrolyte sealing and TiO2 is the best semiconductor material for the DSSC photoanode. Since TiO2 has low electron mobility and has issues regarding light absorption capability, the morphology, operation principles, fabrication techniques, and issues related to the DSSC technology are being studied to help DSSCs become more commercially viable. Band-gap engineering has been introduced for this purpose. Altering the band gap may modulate the electrical and optical properties and size quantization can narrow down the band gap of semiconductor oxides. Various cations have been tested to improve light absorption capability and electron mobility. Cations have also been employed to modify the electronic and optical properties of TiO2 [4]
New oxide materials have been extensively performed to improve the electronic structure and surface properties of the photoanodes, such as nitrogen, carbon, sulfur, and fluorine were found to enhance the absorption of visible light. The performance of the solar cells using N doped TiO2was also studied. The effect of aliovalent doping on DSSCs is correlated to the thermodynamic stability of oxygen vacancy, which is common in TiO2[6].
Figure 3: The performance DSSCs using oxide semiconductors other than TiO2 [6].
The inverter is a crucial component of a solar energy system. The photovoltaic industry has been developing reliability, efficiency, monitoring options, and cost and weight reduction in the arena of inverter technology. In the late nineties, grid-paralleled inverters in the two to fifteen-kilowatt range had no more than three manufacturers and four of five UL-listed models available for the design engineer. There are ten or more manufacturers with over two dozen models in this range (examples include: Beacon Power, Magnetek, Fronius, SMA, Sharp, Xantrex, Solectria, PV Powered, Outback, and Vanner). Out of the most exciting inverter technologies, there have been the latest advancements in Inverter technology.
String Inverters: These inverters are able to handle electricity produced by multiple solar panels, and are very cost-efficient and cheaper than micro inverters. Solar Inverters from 2010 consisted of communications cards, dataloggers, modems, and other accessories and were limited in their design flexibility and software capabilities. Manufacturers came up with software that mitigates the shade more efficiently and without the need for additional hardware. Modern string inverters also have integrated arc fault detection, reducing the risk of arcing in modules or connectors. It also consists of anti-Islanding protection, ground fault protection, insulation monitoring, and easy field serviceability [7]
Figure 4: TMEIC’s Solar Ware Ninja modular string inverter skid [8].
Small molecule organic semiconductors: Organic semiconductors are promising because of their low-cost fabrication, their chemical and electrical properties can be significantly tuned, efficiently manufactured, and low environmental impact compatibility with flexible substrates. The electronic conductivity of OSCs lies between metals and insulators, spanning a broad range between 10-7 and 103 Scm-1. These materials are either based on oligomers such as pentacene, anthracene, rubrene, or oligothiophenes or on polymers such as polypyrrole, polyacetylene, poly(3-hexylthiophene) (P3HT), or poly(p-phenylene vinylene) [9]. OSCs are currently entering mass production. They are attractive for building-integrated photovoltaics since their light weight and flexibility allow for easy mounting of the modules onto roofs and facades. Two processing techniques were established to make OSCs more efficient: 1) dry processing (thermal evaporation) for planar-heterojunction (PHJ) and bulk-heterojunction (BHJ) solar cells and 2) solution processing (spin-coating, inkjet printing, dip-coating, spraying technique) for BHJ solar cells. Record efficiencies have independently been reported for tandem small-molecule/oligomer solar cells produced by controlled thermal evaporation of the various layers [9]. Current efforts are being made to improve the processability and tunability of organic materials. Further exploration of structure-property correlations in the context of device efficiency as well as durability can facilitate the widespread utilization of OSCs. Such as organic photovoltaics, Organic Light-emitting Diodes, and Organic thin film transistors.
Changing energy costs over the past few years
Even though improvements have been made in modern solar panels, making solar energy more efficient and continuous, there hasn’t been an increase in production cost in the solar industry. From 2015 to 2020, the cost per watt of solar power plummeted from $2.24 to $1.25, nearly half the cost. Ten years ago, the price per watt was a staggering $5.79. In 2016, the average installation cost for small business and residential purposes was $2.06/Watt and fell to $1.89/Watt in 2021. Reducing costs means that solar panels pay for themselves much quicker than ever before, all while reducing dependence on fossil fuels.[2] The low costs have enabled solar tech industries to expand into new markets and deploy thousands of systems nationwide. An average-sized residential system has dropped from a pre-incentive price of $40,000 in 2010 to roughly $25,000 today, while recent utility-scale fees range from $16/MWh - $35/MWh, competitive with all other forms of generation [10].
Figure 5: US solar PV pricing trends and deployment growth [10]
Future directions of solar technology
Photovoltaics research is ongoing to improve solar panels and create an efficient energy supply and storage system to help reduce the dependency on fossil fuels. Different metal oxides are also being tested for DSSCs and incorporating new materials into OSCs to make these devices more commercially viable. The expected future direction towards solar technology contains: having buildings built to combine an energy-efficient design, construction practices, and renewable energy technologies for a net-zero energy building. In effect, the building will conserve enough and produce its energy supply to create a new generation of cost-effective buildings with zero net annual need for non-renewable energy[11]. Even though many approaches are being made to lower the cost of solar-powered devices, the high price of solar trash is a matter of concern. The financial incentive to invest in recycling has never been strong in solar. While panels contain small amounts of valuable materials such as silver, they are mostly made of extremely low-value glass, costing around $20–$30 to recycle one panel. Sending that same panel to a landfill would cost a mere $1–$2 [12]. The world could witness some 78 million tons of solar trash disposed in landfills and other waste facilities by 2050, according to the International Renewable Energy Agency (IRENA). They predict the U.S. will contribute 10 million metric tons of trash to the 2050 total [13]. Recently, much attention has been given to creating aesthetically pleasing panels and those that take less space. However, specialized labor is required to remove these advanced panels. This can mean more jobs because, in 2022, more than 263,000 people were working in solar at more than 10,000 companies in every U.S. state before there was a concern to recycle/clean up solar trash. The solar industry generated nearly $35 billion of private investment in the American economy [10]. Researchers are addressing these issues by making solar energy storage more efficient by implementing organic-based solar cells instead of silicon-based ones.
Solar Waste
Solar waste is hazardous due to the amount of small metals the panels contain. Recently solar waste has been bought to light, and researchers are finding a way to recycle solar panels. A crystalline silicon solar technology will be implemented, constructed with an aluminum frame, glass, copper wire, polymer layers, back sheets, silicon cells, and a plastic junction box. The polymer layers seal the panel from exposure to weather but can make recycling and panel disassembling difficult, as high temperatures are often required to loosen the adhesive [14]. Different solar panels include the removal of the frame and junction box; separation of the glass and the silicon wafer through thermal, mechanical, or chemical processes; and separation and purification of the silicon cells and specialty metals (e.g. silver, tin, lead, copper) through chemical and electrical techniques. It is important to recycle solar panels in an ideal way and the steps include removing the frame and junction box, separating the glass and silicon wafer, and separation or purification of silicon cells and other metals/materials. Another way to reduce solar waste is by reusing solar panels or refurbishment them and including them in electric bikes, vehicle charging stations, or other remote locations.
Figure 6: Crystalline-silicon solar technology [14].
Conclusion
Nowadays, the usage of fossil fuels and greenhouse gas emissions has increased tremendously. Global carbon emissions from fossil fuels have risen dramatically since the industrial revolution began. Since 1970, CO2 emissions have increased by about 90%, with emissions from fossil fuel combustion and industrial processes contributing about 78% of the total greenhouse gas emissions increase from 1970 to 2011 [14]. The emergence of transitioning into using renewable energy and emitting less greenhouse gases has been a concern for a long time. Harnessing solar power has a long history that dates to thousand years ago. Primitive applications have come a long way since then and have taken a sophisticated approach toward maximizing the harnessed energy and devising various applications through advanced technology [3]. Dye-sensitized solar cells, small molecule organic semiconductors, and Inverter technology seem promising in harnessing solar power more efficiently and using lower manufacturing costs. There are concerns about solar waste and having significant increase over the past few years, but different strategies are being tested to recycle solar trash. Solar energy evolution means components are cheaper than ever, produce more power, and have a smaller footprint than five years ago. Solar power has experienced continuous growth and will continue to do so in the upcoming years. It has developed the potential to address energy poverty and provide energy to regions without grid infrastructure. Out of all renewable sources, solar power will become more dominant due to its clean, renewable energy production and contribute to a more sustainable world.
About Authors
Dr. Raj Shah is a Director at Koehler Instrument Company in New York, where he has worked for the last 28 years. He is an elected Fellow by his peers at IChemE, CMI, STLE, AIC, NLGI, INSTMC, Institute of Physics, The Energy Institute and The Royal Society of Chemistry. An ASTM Eagle award recipient, Dr. Shah recently coedited the bestseller, “Fuels and Lubricants handbook”, details of which are available at ASTM’s Long-Awaited Fuels and Lubricants Handbook 2nd Edition Now Available (https://bit.ly/3u2e6GY). He earned his doctorate in Chemical Engineering from The Pennsylvania State University and is a Fellow from The Chartered Management Institute, London. Dr. Shah is also a Chartered Scientist with the Science Council, a Chartered Petroleum Engineer with the Energy Institute and a Chartered Engineer with the Engineering council, UK. Dr. Shah was recently granted the honourific of “Eminent engineer” with Tau beta Pi, the largest engineering society in the USA. He is on the Advisory board of directors at Farmingdale university (Mechanical Technology) , Auburn Univ ( Tribology ), SUNY, Farmingdale, (Engineering Management) and State university of NY, Stony Brook ( Chemical engineering/ Material Science and engineering). An Adjunct Professor at the State University of New York, Stony Brook, in the Department of Material Science and Chemical engineering, Raj also has over 590 publications and has been active in the energy industry for over 3 decades.
Ms.Mrinaleni Das and Ms. Salowa Siddique are part of a thriving renewable energy internship program at Koehler Instrument company in Holtsville, and are students of Chemical Engineering at Stony Brook university, Long Island, NY where Dr. Shah is the current chair of the external advisory board of directors.
References
1.Crippa, Monica & Guizzardi, Diego & Solazzo, Efisio & Muntean, M. & Schaaf, Edwin & Monforti, Fabio & Banja, Manjola & Olivier, Jos & Rossi, Simone & Vignati, E.. (2021). GHG emissions of all world countries booklet 2021 report. 10.2760/173513.
2. How Solar Panels and Solar Energy Have Evolved over the Past 5 Years. https://www.renogy.com/blog/how-solar-panels-and-solar-energy-have-evolved-over-the-past-5-years/ (accessed 2023-06-28).
3. Tabassum, S., Rahman, T., Islam, A. U., Rahman, S., Dipta, D. R., Roy, S., Mohammad, N., Nawar, N., & Hossain, E. (2021). Solar Energy in the United States: Development, Challenges and Future Prospects. Energies, 14(23), 8142. https://doi.org/10.3390/en14238142
4.https://solarsena.com/different-types-solar-cells-efficiencies/
5. Shakeel Ahmad, M., Pandey, A., & Abd Rahim, N. (2017). Advancements in the development of TiO2 photoanodes and its fabrication methods for dye-sensitized solar cell (DSSC) applications. A review. Renewable and Sustainable Energy Reviews, 77, 89-108. https://doi.org/10.1016/j.rser.2017.03.129
6.Lee, J., & Yang, M. (2011). Progress in light harvesting and charge injection of dye-sensitized solar cells. Materials Science and Engineering: B, 176(15), 1142-1160. https://doi.org/10.1016/j.mseb.2011.06.018
7.The-Evolution-of-String-Inverters-09-24-21, www.fronius.com/en-us/usa/solar-energy/installers-partners/info-center/blog/the-evolution-of-solar-string-inverters. Accessed 6 Aug. 2023.
8.https://www.solarpowerworldonline.com/2021/08/efficiencies-utility-scale-solar-inverter-design-developer-options/
9.Mishra, A., & Bäuerle, P. (2012). Small Molecule Organic Semiconductors on the Move: Promises for Future Solar Energy Technology. Angewandte Chemie International Edition, 51(9), 2020-2067. https://doi.org/10.1002/anie.201102326
10.Solar Industry Research Data. https://www.seia.org/solar-industry-research-data (accessed 2023-07-01).
11.https://www1.eere.energy.gov/solar/pdfs/solar_timeline.pdf (accessed 2023-06-29).
12.“The Dark Side of Solar Power.” Harvard Business Review, 19 Apr. 2022, hbr.org/2021/06/the-dark-side-of-solar-power.
13. Kennedy, Ryan. “Solar Trash: Without Intervention, a Shocking (and Costly) Amount Will Be Produced.” Pv Magazine USA, 29 June 2021, pv-magazine-usa.com/2021/06/29/solar-trash-without-intervention-a-shocking-and-costly-amount-will-be-produced/.
14. EPA, www.epa.gov/hw/solar-panel-recycling. Accessed 12 July 2023.
The content & opinions in this article are the author’s and do not necessarily represent the views of AltEnergyMag
Comments (0)
This post does not have any comments. Be the first to leave a comment below.
Featured Product
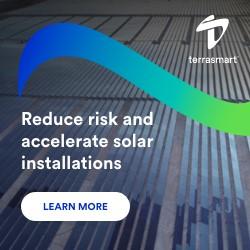